Table of Contents
Introduction to Steel Beams(Mild Steel)
Steel beams are long, sturdy pieces of steel that bear loads and provide support in construction. They are essential for creating frameworks for buildings, bridges, and other infrastructure. Due to their strength, flexibility, and durability, steel beams are a preferred choice in modern construction.
Steel beams are not just structural components; they are the very backbone of modern construction. From skyscrapers to bridges, warehouses to residential buildings, steel beams provide essential support and strength. This article explores ten powerful facts, types, specification, application and more about steel beams like
steel beams types, Steel i beam weight chart, section classification, Lateral Stability of beam ,Lateral torsion buckling ,support condition ,effective length , cantilever beams ,buckling of real beam, plasticity effect , Behavior of beams in bending, steel beams in flexural, Yielding and plastic Behavior and much more, highlighting their significance in today’s architecture and engineering. So lets start..
10 Powerful Facts About Steel Beams
*Strength and Durability
Steel beams are renowned for their exceptional strength and durability. Unlike wood or concrete, steel has a high strength-to-weight ratio, making it ideal for supporting heavy loads over long spans. This characteristic allows architects and engineers to design structures with fewer columns and more open spaces, maximizing usable floor area.
*Versatility in Design
One of the most significant advantages of steel beams is their versatility in design. They can be fabricated into various shapes and sizes, such as I-beams, H-beams, T-beams, and C-beams, to suit different structural requirements. This flexibility enables creative and efficient solutions in construction projects, accommodating diverse architectural designs and engineering needs.
*Fire Resistance
Contrary to popular belief, steel beams exhibit excellent fire resistance when properly protected. They do not burn or contribute to the spread of flames, unlike wood or some other building materials. In high-rise buildings and structures where fire safety is crucial, steel beams provide a reliable and safe option for structural support.
*Sustainability and Recyclability
MS beams are highly sustainable and environmentally friendly. Steel is one of the most recycled materials globally, with a recycling rate exceeding 90%. Using recycled steel in construction significantly reduces energy consumption and greenhouse gas emissions compared to producing new steel from raw materials. Moreover, at the end of a building’s life cycle, steel can be recycled indefinitely without losing its quality or strength.
*Speed of Construction
MS beams contribute to faster construction times compared to traditional building materials. Prefabricated steel beams can be manufactured off-site and quickly assembled on-site, reducing construction timelines and labor costs. This efficiency is particularly advantageous in urban areas and environments where time constraints are critical.
*Longevity and Maintenance
MS beams are known for their longevity and minimal maintenance requirements. They are resistant to pests, mold, and decay, which can be significant issues with organic materials like wood. Properly maintained steel structures can withstand harsh weather conditions and environmental factors, ensuring durability and structural integrity over decades.
*Cost Effectiveness
Despite its initial higher cost compared to some traditional materials, MS beams offer long-term cost-effectiveness. Their strength and durability reduce the need for frequent repairs and replacements, saving money on maintenance and extending the lifespan of buildings and infrastructure. Additionally, the speed of construction associated with steel beams can result in overall cost savings during the project’s lifecycle.
*Structural Performance
MS beams provide superior structural performance in various applications, including buildings, bridges, industrial facilities, and residential structures. They offer high tensile strength, allowing for longer spans between supports and fewer columns, thereby enhancing architectural flexibility and design possibilities. This capability is crucial in creating open, airy spaces that meet modern aesthetic and functional requirements.
*Sustainable Building Practices
Incorporating steel beams into construction aligns with sustainable building practices and green building certifications. Steel’s recyclability, energy efficiency, and durability contribute to LEED (Leadership in Energy and Environmental Design) and other green building rating systems’ criteria. By choosing steel beams, architects and developers demonstrate a commitment to reducing environmental impact and promoting sustainable development practices.
*Innovative Applications
The use of steel beams continues to evolve with advancements in technology and engineering. Innovations such as composite steel beams, which combine steel with other materials like concrete, offer enhanced structural performance and efficiency. Additionally, developments in fabrication techniques, such as laser cutting and 3D modeling, improve precision and customization in steel beam manufacturing, further expanding their applications in complex architectural projects.
Types of MS Beams
I-Beam (H-Beam)
- Shape: Resembles the letter “I” or “H.”
- Usage: Commonly used in residential and commercial buildings, bridges, and frames.
- Characteristics: Strong load-bearing capacity, high resistance to bending
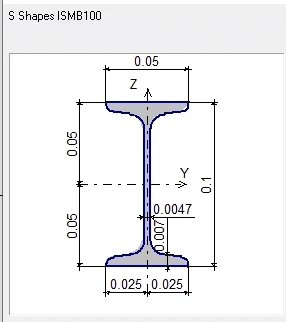
T-Beam
- Shape: Resembles the letter “T.”
- Usage: Used in reinforced concrete, bridges, and roof structures.
- Characteristics: Effective for tensile strength, often used where a flat, broad surface is required.

C-Beam (Channel Beam)
- Shape: Resembles the letter “C.”
- Usage: Used in construction for purlins, side rails, and floor systems.
- Characteristics: Good for supporting load and providing stability.
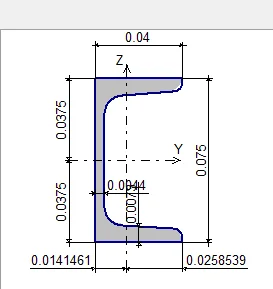
L-Beam (Angle Beam)
- Shape: Resembles the letter “L.”
- Usage: Used for supporting corners and edges.
- Characteristics: Provides support and reinforcement, useful for framing and trimming.
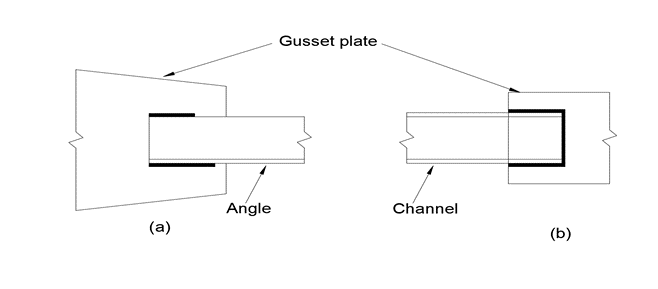
Steel Beam Section Classification
Steel beams are classified based on their ability to withstand bending without local buckling. This classification is crucial for understanding the behavior of steel beams under different loading conditions. The classification is divided into four categories:
Plastic (Class 1), Compact (Class 2), Semi-Compact (Class 3), and Slender (Class 4). These categories depend on the yield and plastic moments along with the rotation capacities of the sections.
Local Buckling
Local buckling occurs when a part of the beam’s cross-section (like the flange or web) buckles under compression before the entire section yields. Proper classification helps engineers choose the right type of beam for different structures to prevent local buckling and ensure safety.
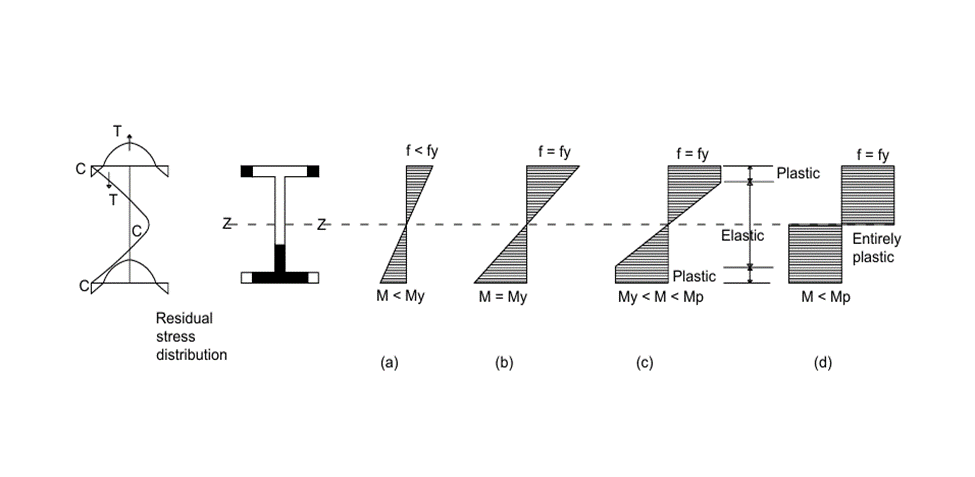
Note : Shaded portion indication that have reached fy due to residual stress of stage b.
Stress distribution at different stages of loading
Classification Categories
Plastic (Class 1):
- Description: These sections can form plastic hinges and sustain large rotations without any local buckling.
- Yield and Plastic Moment: The section can reach its full plastic moment capacity (the maximum moment it can resist) and maintain it during significant rotations.
- Rotation Capacity: Very high, allowing for large deformations without losing strength.
- Usage: Suitable for structures requiring significant ductility, such as earthquake-resistant buildings.
Compact (Class 2):
- Description: These sections can reach their full plastic moment but have a limited rotation capacity before local buckling occurs.
- Yield and Plastic Moment: Can achieve the plastic moment capacity but with less rotation compared to Class 1.
- Rotation Capacity: Moderate, providing some level of ductility but less than Class 1.
- Usage: Used in structures where some ductility is needed, but large rotations are not expected.
Semi-Compact (Class 3):
- Description: These sections can reach their yield moment (the moment at which the material starts to deform plastically) but cannot reach the full plastic moment because local buckling occurs first.
- Yield and Plastic Moment: Can only reach the yield moment, not the plastic moment.
- Rotation Capacity: Limited, as local buckling occurs shortly after yielding.
- Usage: Suitable for structures where high ductility is not crucial but strength is still needed.
Slender (Class 4):
- Description: These sections experience local buckling before reaching the yield moment.
- Yield and Plastic Moment: Cannot achieve the yield moment due to premature local buckling.
- Rotation Capacity: Very low, as local buckling limits the section’s ability to deform plastically.
- Usage: Typically used in less critical structures where weight savings are more important than ductility and strength.
Classification | Description | Moment Capacity | Rotation Capacity | Usage |
---|---|---|---|---|
Plastic (Class 1) | High rotation capacity, full plastic moment | Full Plastic Moment | Very High | Used in high-ductility structures |
Compact (Class 2) | Moderate rotation capacity, full plastic moment | Full Plastic Moment | Moderate | Used in moderately ductile structures |
Semi-Compact (Class 3) | Limited rotation capacity, yield moment only | Yield Moment Only | Limited | Used where ductility is less important |
Slender (Class 4) | Very low rotation capacity, local buckling before yield moment | Less than Yield Moment | Very Low | Used in weight-sensitive structures |
“Unlocking Welded Connections and welding: Top 10 Dynamic Techniques for Steel Structures”
Lateral Stability Of beam
Lateral stability of a beam refers to its ability to resist bending and twisting when subjected to transverse loads. Transverse loads are forces applied perpendicular to the length of the beam, such as the weight of a structure or environmental forces like wind.
To understand the lateral stability of a beam, it’s essential to consider two important assumptions about ideal beams:
Assumption 1: The Compression Flange of the Beam is Restrained from Moving Laterally
The first assumption for ensuring lateral stability is that the compression flange of the beam is prevented from moving sideways. The compression flange is the part of the beam under compressive stress when the beam bends. If this flange can move laterally, the beam might buckle or twist, leading to structural failure. Restraining the compression flange can be achieved through bracing or connecting it to other structural elements, ensuring it remains stable under load.
Assumption 2: Any Form of Local Buckling is Prevented
The second assumption is that any form of local buckling is prevented. Local buckling occurs when a small section of the beam deforms under stress, which can compromise the overall stability of the structure. Preventing local buckling involves using thicker materials, adding stiffeners, or ensuring proper support along the beam’s length. This assumption ensures that the beam maintains its shape and strength, even under significant transverse loads.
Lateral Torsion Buckling of Symmetric Section
Lateral torsion buckling is a type of instability that can occur in beams, particularly those with symmetric sections like I-sections, when they are subjected to bending. This instability happens when a beam deflects laterally and twists simultaneously under load. Understanding how lateral torsion buckling works is essential for ensuring the structural integrity of beams in construction and engineering projects.
How Does a Beam Fail in Lateral Torsion Buckling?
When a beam is loaded, it is primarily supposed to bend in the direction of the applied load. However, if the beam’s slenderness (length to width ratio) is high, and if it is not properly braced, it may experience lateral torsion buckling. This failure mode involves the beam bending sideways (laterally) and twisting around its longitudinal axis (torsion).
Here’s how the process typically unfolds:
- Initial Loading: As the load is applied, the beam starts to bend.
- Instability: If the load reaches a critical point, the beam cannot withstand the bending moment and begins to move sideways.
- Buckling: The beam then twists along its length, leading to a combined lateral and torsional deformation.
- Failure: The beam can no longer support the load, resulting in structural failure.
Assumptions for I-Section in Lateral Torsion Buckling
When analyzing lateral torsion buckling for I-sections, several assumptions are usually made:
- Symmetry: The I-section is symmetric about its major and minor axes, which simplifies the analysis.
- Elastic Behavior: The material remains elastic, meaning it returns to its original shape after the load is removed, up until the point of buckling.
- Uniform Cross-Section: The cross-section of the beam is uniform along its length.
- Straight and Prismatic: The beam is straight and has a consistent shape along its length.
- No Initial Imperfections: The beam is assumed to be perfect without initial deformations or flaws.
- Constant Loading: The load is applied gradually and remains constant.
Role of Torsion in Lateral Torsion Buckling
Torsion plays a critical role in the phenomenon of lateral torsion buckling. Here’s how:
- Twisting: Torsion causes the beam to twist around its longitudinal axis. This twisting can lead to a significant reduction in the beam’s load-carrying capacity.
- Interaction with Lateral Deflection: As the beam twists, it also deflects laterally. The combination of these two movements results in a buckling mode that is more complex than simple bending.
- Critical Load: The presence of torsion lowers the critical load at which the beam buckles. This means the beam can fail under a lower load than if it were only bending.
- Stabilization: Proper bracing and support can counteract the effects of torsion, stabilizing the beam and preventing lateral torsion buckling.
lateral torsion buckling is a critical consideration for the stability of beams with symmetric sections, like I-sections. It involves the beam bending sideways and twisting under load, leading to potential structural failure. Understanding the assumptions and the role of torsion in this process helps in designing safer and more reliable structures.
Factors Affecting Lateral Stability
Lateral stability in structures is crucial to prevent sideways movement or bending, especially in beams. Several factors influence this stability:
1. Influence of Cross-Sectional Shape on Lateral Torsion Buckling
The shape of a beam’s cross-section significantly impacts its resistance to lateral torsion buckling. Beams with wider flanges, like I-beams, are generally more stable because they have higher resistance to twisting. Conversely, narrow or slender beams are more prone to buckling under lateral loads.
2. Support Condition
The way a beam is supported affects its lateral stability. Fixed supports provide greater resistance to movement and rotation, enhancing stability. Simply supported or pinned ends, on the other hand, offer less resistance, making the beam more vulnerable to lateral instability.
rmediate braces are used to improve a beam’s lateral stability by providing additional support points along its length.
- a) Torsional Bracings: These braces prevent the beam from twisting by restraining the torsional movement. They connect to the beam at specific points, enhancing stability.
- b) Continuous Torsional Bracings: These braces provide continuous support along the length of the beam, preventing lateral and torsional movements throughout the entire beam.
4. Cantilever Beam
Cantilever beams, which are fixed at one end and free at the other, face unique challenges in lateral stability. The free end can easily move or twist under load, making it more susceptible to buckling. Proper design and support are essential to maintain stability in cantilever beams.
5. Effective Length
The effective length of a beam is the distance between points where the beam is restrained against
lateral movement. A longer effective length increases the risk of lateral torsion buckling, while a shorter effective length enhances stability. Properly calculating and minimizing the effective length is crucial for ensuring lateral stability in beams.
By considering these factors—cross-sectional shape, support conditions, intermediate braces, cantilever beam design, and effective length—engineers can design structures that maintain lateral stability and resist buckling under various loads.
MS Beam Specifications
Steel beams come in various sizes and grades, depending on the requirements of the construction project. Below is a table showing common specifications for steel beams used in India and the US.
Specification | India (IS 2062) | US (ASTM A36) |
---|---|---|
Grade | E250, E350, E410 | A36 |
Tensile Strength | 410-490 MPa | 400-550 MPa |
Yield Strength | 250-410 MPa | 250 MPa |
Common Sizes | ISMB 100, ISMB 200, ISMB 300…… | W10x12, W14x30, W24x55…. |
Dimensions | Varies (100 mm to 600 mm depth) | Varies (6 inches to 24 inches depth) |
Applications of MS Beams
- Buildings:
- Residential: Used in constructing houses, providing structural support for floors and roofs.
- Commercial: Used in skyscrapers, office buildings, and shopping malls.
- Bridges:
- Steel beams provide the main support structure for bridges, ensuring stability and load distribution.
- Industrial Structures:
- Used in factories, warehouses, and other industrial buildings for their strength and durability.
- Infrastructure:
- Used in the construction of roads, railways, and other infrastructure projects.
Design Considerations
When designing structures with steel beams, engineers must consider several factors:
- Load-Bearing Capacity: Calculating the maximum load a beam can support.
- Span Length: Determining the distance a beam can span without additional support.
- Deflection: Ensuring the beam does not bend excessively under load.
- Connection Methods: Deciding how beams will be connected to other structural elements (welding, bolting, etc.)
Conclusion
Steel beams are a vital component in the construction industry, providing essential support for a variety of structures. Understanding the different types, specifications, and applications of steel beams helps in making informed decisions for construction projects. Both Indian and US standards ensure that steel beams used in construction are reliable and meet specific performance criteria.
Discover more from
Subscribe to get the latest posts sent to your email.
6 thoughts on ““10 Powerful Facts About Steel Beams: The Backbone of Modern Construction””
Comments are closed.